|
|
Developmental biology - Mammary Duct Formation
3D Computer Model of Breast Duct Formation
Engineers recreate the first 3D computer model of how tiny tubes funnel milk through breasts...
Working with hundreds of time-lapse videos of mouse tissue, a team of biologists joined up with civil engineers to create what is believed to be the first 3D computer model to show precisely how tiny tubes form to funnel milk through breasts of mammals.
A report on these cellular models was published in Developmental Cell. Creating the model employed science and engineering principles commonly used to predict forces at work on bridges and buildings — while "miniaturizing" them for cells.
Understanding how milk ducts form, the team believes they may also be lending insight into how diseases such as metastatic breast cancers spread throughout the body.
"We learned from this study that cancers would not need to 'invent' cell migration, but only reactivate dormant — but normal — duct migration programs, in the wrong place and wrong time," says Andrew Ewald PhD, professor of cell biology at the Johns Hopkins University School of Medicine and a researcher at the Johns Hopkins Kimmel Cancer Center.
Ewald emphasizes that the computer model was created through observations of mouse tissue grown in the laboratory. But, as mammalian biology is so highly conserved across species, the same processes are likely applicable to most, if not all mammals.
Ewald's lab worked with a research team from the University of Waterloo in Canada led by Wayne Brodland, Department of Civil and Environmental Engineering and Centre for Bioengineering and Biotechnology, to build the computer model. The team compared how migrating cells interact with their neighbors and organized into "scaffolding," as they would with systems of beams that support man-made structures.
"This was an interesting structural engineering problem to solve," says Matthew Perrone, a civil engineering master's student at the Johns Hopkins Whiting School of Engineering. "In addition to determining the forces acting within a finished duct that hold it together, we had to compute the forces that allow it to support itself while still developing."
As the mouse mammary tissues grew breast milk ducts, the team measured all angles between one cell border and the next, using this info to calculate relative strength and direction of forces acting on each cell in ductal tissue.
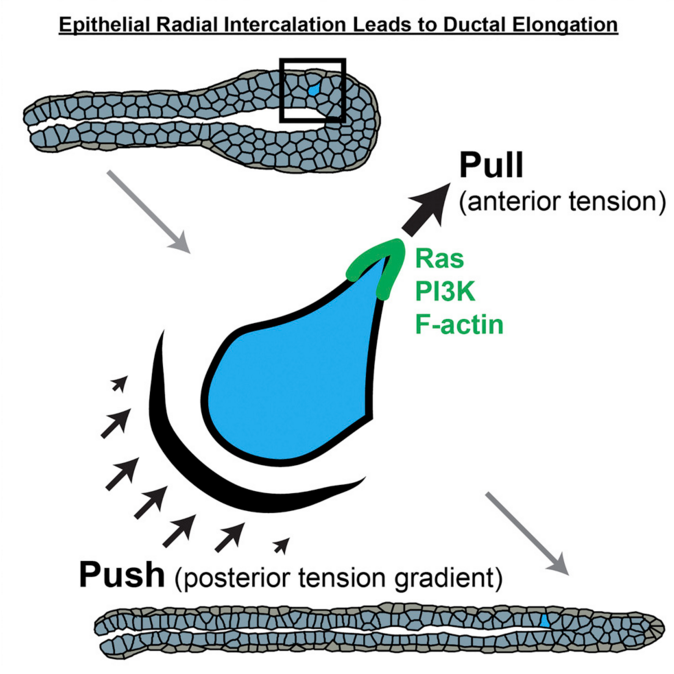
These cellular scaffolds look similar to a basketball net, suggests Ewald.
"Imagine an invisible hand pulling on the net, in which the angles between all of the links change, such that you can predict where the hand is and what it's doing."
These angles change as a cell moves through tissue, a process incited by chemical gradients within a cell. Using fluorescent molecules to mark these chemicals, the research team found the signaling proteins RAS, phosphatidylinositol 3-kinase and F-actin, concentrate in the front of a cell. They watched as these signals prompted the cell to change from a typical shapeless epithelial cell into a unique teardrop shape as it began to move.
"After seeing this occur hundreds of times, we knew that [teardrop] shape must be important for the biology and the mechanics of the system," says Neil Neumann, lead author on the study and an MD/PhD candidate at the Johns Hopkins University School of Medicine.
That teardrop shape, researchers found, was the result of a pulling force at the front of the cell, and a pushing force at the back. These same forces are at work in single cells as each moves about outside of tissues.
"Almost all of cell migration research over the past 50 years has been based on single cells that have been removed from a normal context and placed on a rigid surface such as a petri dish. Our results reassure us that our understanding of the forces at work on a simple system like a single cell can be applied to more complex environments."
Andrew J. Ewald PhD, Department of Cell Biology and Center for Cell Dynamics, School of Medicine, Johns Hopkins University, Baltimore, Maryland, USA.
However, Ewald adds, a major difference between a cell moving in isolation and one moving through complex living tissue is that in tissue, a cell must form connections with neighboring cells in order to move at all. Ewald's team saw that cells at the front of an elongating duct replicate layer after layer of daughter cells directly below them, like a ball slowly filling from the outside inward. To elongate a duct, cells from the lower layers crawl up to the surface, expanding the surface area and the length of the duct.
With all of this information to encode, the initial cellular scaffolding model was incomplete. Structures sprawled in all directions, rather than growing directionally into a long, linear tube typical to healthy mammary ducts.
"This failure told us that for normal tubes to form, we needed some other force at the edges of a tube," Ewald explains.
Ultimately, researchers found how stationary cells in tissue create fibers to fortify that cell's shape. With forces created by these fibers were added to the model, the tubes finally elongated.
"It is similar to blowing up a balloon inside of a paper tube. The rigid outer layer directs the expansion," says Ewald.
Researchers believe this computer model will enable more mult-idisciplinary studies on how cells migrate in healthy organs, thus providing insight into how diseased cells, such as cancers, may use these processes to spread.
"An exciting part of this interdisciplinary work is that each side of the project informed and enhanced the work of the other," says Neumann. "The experiments that we performed improved the computer model, and the results from the model gave us new insights into how the biology worked."
Highlights
• Cells in epithelial tissues display a similar migratory logic as single cells
• Migration through tissues requires specific interfacial tension ratios
• Intercalation at the tissue surface couples migration to tube elongation
• Molecular signaling coordinates with cell and tissue mechanics to elongate tubes
Summary
We sought to understand how cells collectively elongate epithelial tubes. We first used 3D culture and biosensor imaging to demonstrate that epithelial cells enrich Ras activity, phosphatidylinositol (3,4,5)-trisphosphate (PIP3), and F-actin to their leading edges during migration within tissues. PIP3 enrichment coincided with, and could enrich despite inhibition of, F-actin dynamics, revealing a conserved migratory logic compared with single cells. We discovered that migratory cells can intercalate into the basal tissue surface and contribute to tube elongation. We then connected molecular activities to subcellular mechanics using force inference analysis. Migration and transient intercalation required specific and similar anterior-posterior ratios of interfacial tension. Permanent intercalations were distinguished by their capture at the boundary through time-varying tension dynamics. Finally, we integrated our experimental and computational data to generate a finite element model of tube elongation. Our model revealed that intercalation, interfacial tension dynamics, and high basal stress are together sufficient for mammary morphogenesis.
Authors: Neil M. Neumann, Matthew C. Perrone, Jim H. Veldhuis, Robert J. Huebner, Huiwang Zhan, Peter N. Devreotes, Wayne Brodland, Andrew J.Ewald.
Acknowledgements
This research was supported by the Natural Sciences and Engineering Research Council of Canada Discovery Grant (RGPIN-36514), the Alexander Graham Bell Master's Canada Graduate Scholarship, the Ontario Graduate Scholarship Program, the American Cancer Society (NIGMS3T32GM007309, NCI U01XA217846 and U54 CA2101732), the National Science Foundation (PD-11-7246), the Johns Hopkins University Catalyst Awards and The Pink Agenda and Breast Cancer Research Foundation.
Return to top of page
| |
|
Jun 19, 2018 Fetal Timeline Maternal Timeline News News Archive
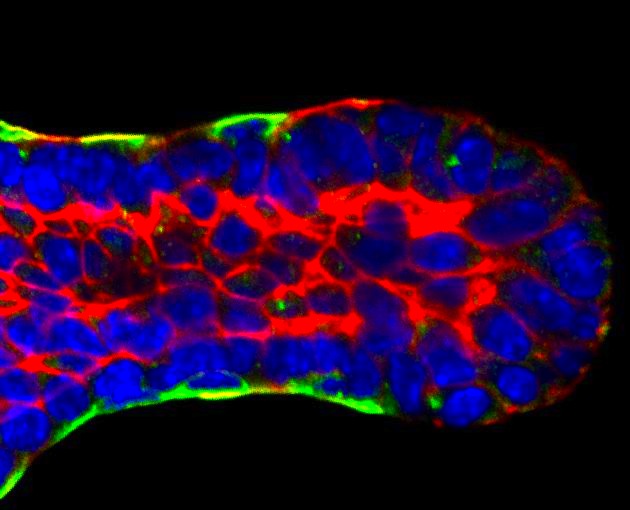 Epithelial tissue cells have similar migratory behavior as single cells. Cell Intercalation on tissue surfaces joins cell migration to tube elongation. Image credit: JHU School of Medicine.
|