|
|
Developmental Biology - Stem Cells
Pressure Helps Stem Cells Heal Bone Breaks
Compressing stem cells around bone break recreates embryonic conditions that help bones heal faster...
Researchers at the University of Illinois at Chicago and the University of Pennsylvania have developed a unique technique using stem cells and flexible implantable bone-stabilizing plates to help speed healing of large bone breaks or defects. The technique allows stem cells applied to break sites to react under mechanical stress, as they do in developing embryos. These forces appear to encourage stem cells to differentiate into cartilage and bone, as well as encourage other cells in the bone to regenerate.
The findings are reported in Science Translational Medicine.
Stem cells that give rise to bone and cartilage are subject to mechanical forces during development and healing, explained Eben Alsberg, the Richard and Loan Hill Professor of Bioengineering and Orthopaedics at the University of Illinois at Chicago and a senior author on the paper.
Stem cells need environmental cues to differentiate into cells that make up unique tissues. When there are large gaps between broken or deformed bones, applying additional stem cells to break sites can help bones heal faster by either actively participating in the regenerative process or stimulating bone formation by neighboring cells.
To use stem cells for bone regeneration, cells need to be delivered to the defect site and differentiate appropriately to stimulate repair. Alsberg and colleagues developed a unique preparation of cells that can be easily handled and manipulated for implantation and that supports the cellular differentiation events that occur in embryonic bone development.
In Alsberg's preparation, stem cells are cultured so that they link to each other to form either sheets or plugs. The preparation also contains gelatin microparticles loaded with growth factors that help the stem cells differentiate. These sheets or plugs can be manipulated and implanted and reduce the tendency for cells to drift away. Alsberg calls these materials "condensates."
In previous studies, Alsberg and colleagues used condensates in a rodent model to help heal bone defects in the skull. They saw that the condensates stayed in place and were able to improve the rate and extent of bone regeneration. More recently, Alsberg teamed up with Joel Boerckel, assistant professor of orthopedic surgery and bioengineering at Penn Medicine and a senior author on the paper, to take the idea one step further.
Boerckel has developed a unique, flexible "fixator." Fixators usually are stiff metal plates or bars used to stabilize bones at break sites. These kinds of fixators minimize the amount of mechanical stress breaks experience as they are healing.
Boerckel's flexible fixator would allow the cells in Alsberg's condensates to experience the compressive forces that are critical for stimulating enhanced cartilage and bone formation.
The researchers used a rat model to determine how the mechanical forces present within bone defects affected the ability of condensates to contribute to bone regeneration. When the researchers used condensate sheets together with a flexible fixator in rats with a defect in their femur, they saw that there was enhanced healing and the bones had better mechanical function compared with control rats that received condensates and stiff, traditional fixators.
"Our findings support the emerging paradigm of 'regenerative rehabilitation,' a concept which marries principles from physical therapy and regenerative medicine. Our goals are to understand how mechanical stimuli influence cell behavior to better impact patient outcomes without additional drugs or devices."
Joel D. Boerckel MD PhD, McKay Orthopaedic Research Laboratory, Department of Orthopaedic Surgery, Perelman School of Medicine, and Department of Bioengineering, University of Pennsylvania, Philadelphia, Pennsylvania; and, Department of Aerospace and Mechanical Engineering, University of Notre Dame, Notre Dame, Indiana; USA.
Abstract
Large bone defects cannot form a callus and exhibit high complication rates even with the best treatment strategies available. Tissue engineering approaches often use scaffolds designed to match the properties of mature bone. However, natural fracture healing is most efficient when it recapitulates development, forming bone via a cartilage intermediate (endochondral ossification). Because mechanical forces are critical for proper endochondral bone development and fracture repair, we hypothesized that recapitulating developmental mechanical forces would be essential for large bone defect regeneration in rats. Here, we engineered mesenchymal condensations that mimic the cellular organization and lineage progression of the early limb bud in response to local transforming growth factor–ß1 presentation from incorporated gelatin microspheres. We then controlled mechanical loading in vivo by dynamically tuning fixator compliance. Mechanical loading enhanced mesenchymal condensation–induced endochondral bone formation in vivo, restoring functional bone properties when load initiation was delayed to week 4 after defect formation. Live cell transplantation produced zonal human cartilage and primary spongiosa mimetic of the native growth plate, whereas condensation devitalization before transplantation abrogated bone formation. Mechanical loading induced regeneration comparable to high-dose bone morphogenetic protein-2 delivery, but without heterotopic bone formation and with order-of-magnitude greater mechanosensitivity. In vitro, mechanical loading promoted chondrogenesis and up-regulated pericellular matrix deposition and angiogenic gene expression. In vivo, mechanical loading regulated cartilage formation and neovascular invasion, dependent on load timing. This study establishes mechanical cues as key regulators of endochondral bone defect regeneration and provides a paradigm for recapitulating developmental programs for tissue engineering.
Authors
Anna McDermott, Devon Mason and Joseph Collins of the University of Pennsylvania; Samuel Herberg and Rui Tang of Case Western Reserve University; Hope Pearson and James Dawahare of the University of Notre Dame; Amit Patwa and Mark Grinstaff of Boston University, and Daniel Kelly of Trinity College Dublin are co-authors on the paper.
Funding
This research was supported by the Naughton Foundation; the Indiana Clinical and Translational Sciences Institute grant 16SDG31230034; the National Science Foundation grant 1435467; National Institute of Arthritis and Musculoskeletal and Skin Diseases grants R01 AR066193, R01 AR063194, and R01 AR069564; National Institute of Biomedical Imaging & Bioengineering grant R01 EB023907; National Institute of Dental and Craniofacial Research grant 5F32DE024712; National Heart, Lung, and Blood Institute award T3HL134622, and Ohio Biomedical Research Commercialization Program award TECG20150782.
Return to top of page.
| |
|
Jun 20 2019 Fetal Timeline Maternal Timeline News
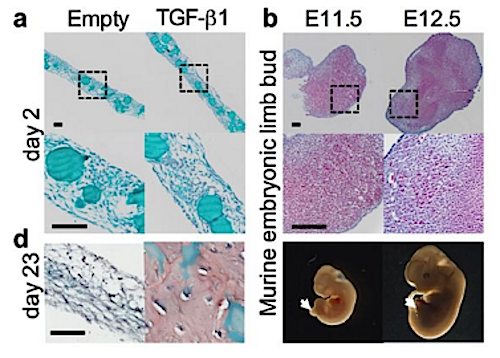 One way bone forms during development is called endochondral ossification — which requires intermediate cartilage proteins. Researchers mimicked this process in rats by implanting cylinders made up of human mesenchymal stem cells with transforming growth factor–ß1, placing all around femur breaks. Using plates to apply pressure, bone bridges formed 4 weeks following stem cell implants demonstrating that mechanical pressure along with tissue engineering can augment bone regeneration. CREDIT The authors.
|